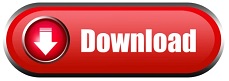
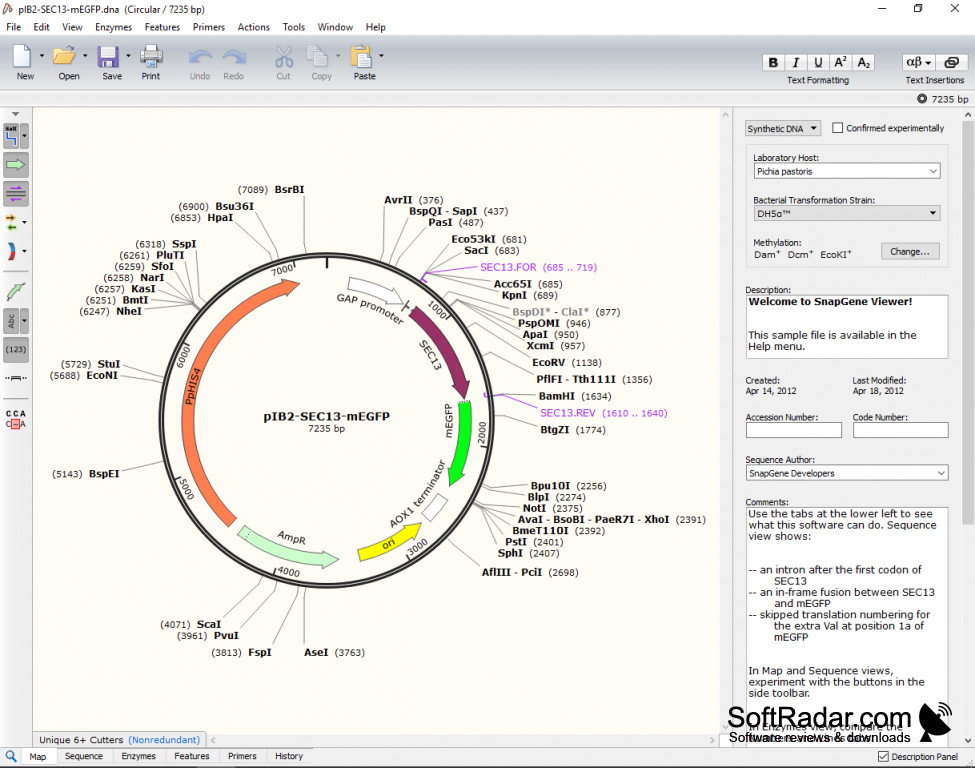
α-SNAP, which is typically encoded by a single gene in animal genomes, binds diverse SNARE complexes and stimulates their disassembly by recruiting and activating NSF ( 1, 11).
Snapgene viewer asterisk free#
SNAREs alone can mediate vesicle fusion in vitro without external energy inputs, but the cis-SNARE complexes formed after fusion must be separated back into free acceptor SNAREs to participate in subsequent fusion events ( 1). Cognate SNAREs on separate membranes promote fusion by bundling together and forming highly stable SNARE complexes that pull the respective membranes together. Eukaryote genomes can encode over 100 different SNARE proteins, with various SNARE subsets generally residing at specific compartments ( 1). SNARE (soluble NSF attachment protein receptor) proteins mediate vesicle fusion ( 1). glycines) resistance was not known ( 8– 10). The discovery that the Rhg1 α-SNAPs carry nonconsensus amino acids at widely conserved C-terminal positions of known importance was intriguing, but a mechanism by which these α-SNAPs contribute to Rhg1-mediated soybean cyst nematode ( H. The soybean ( Glycine max) Rhg1 (resistance to Heterodera glycines 1) locus, one of the most economically important disease resistance loci of any major food crop, carries multiple repeat copies of a gene encoding the major vesicular trafficking chaperone α-SNAP ( 1, 8). Host and pathogen proteins can intervene to alter the course of this traffic to the benefit of the host or the pathogen ( 6, 7). Vesicle trafficking has been most deeply studied in yeast and in neuronal synapses, but is understood in detail for numerous biological systems, including immunity and host–pathogen interactions ( 2– 5).
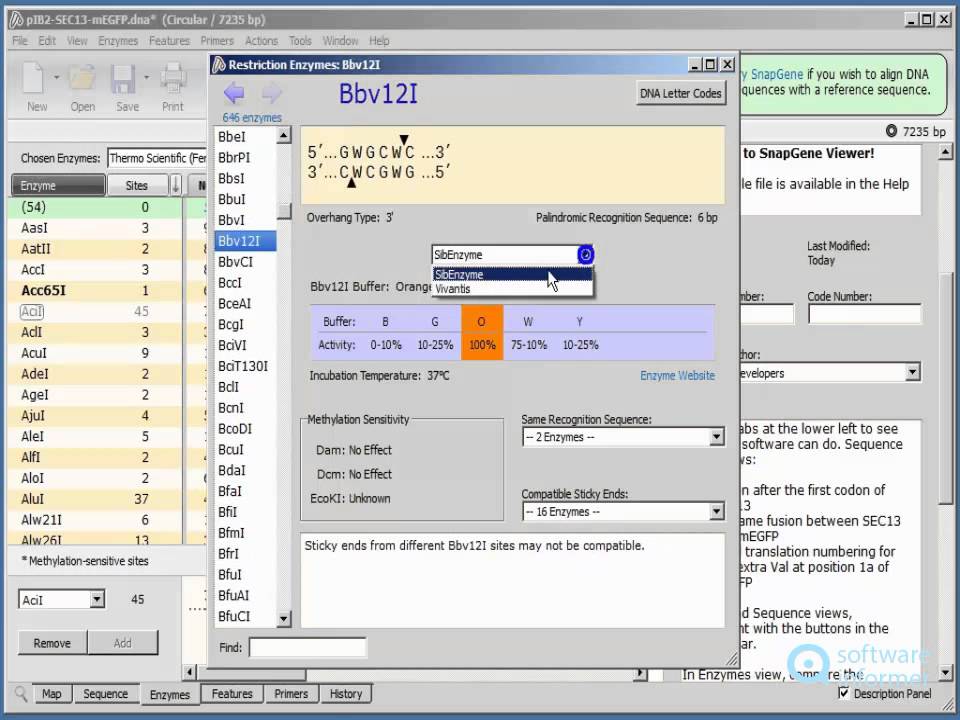
The paradigm of disease resistance through a dysfunctional variant of an essential gene may be applicable to other plant–pathogen interactions.Ī dynamic endomembrane system is a universal trait of eukaryotic cells that enables the transfer of vesicular cargoes throughout the cell and with the cell exterior ( 1). Immunoblots and electron microscopy immunolocalization revealed that resistance-type α-SNAPs specifically hyperaccumulate relative to wild-type α-SNAPs at the nematode feeding site, promoting the demise of this biotrophic interface.
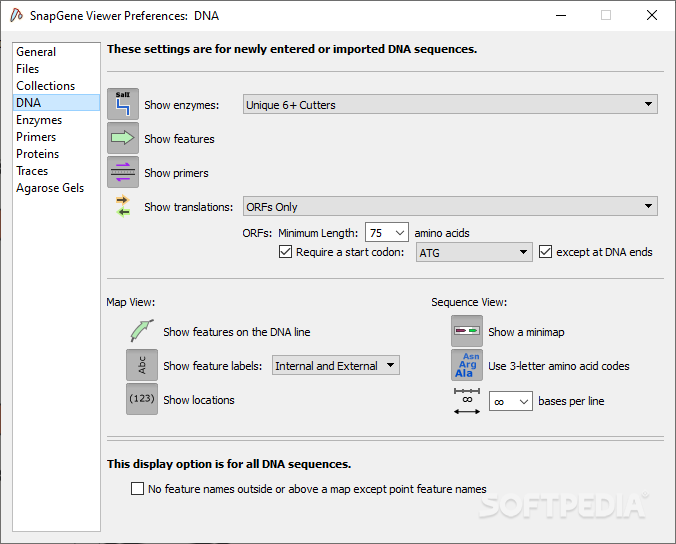
For successful growth and reproduction, SCN dramatically reprograms a set of plant root cells and must sustain this sedentary feeding site for 2–4 weeks. Expression of these α-SNAPs counteracted the cytotoxicity of resistance-type Rhg1 α-SNAPs. Soybean, due to ancient genome duplication events, carries other loci that encode canonical (wild-type) α-SNAPs. Elevated in planta expression of resistance-type Rhg1 α-SNAPs depleted the abundance of SNARE-recycling 20S complexes, disrupted vesicle trafficking, induced elevated abundance of NSF, and caused cytotoxicity. In the present study, we found that the resistance-type Rhg1 α-SNAP is defective in interaction with NSF. Rhg1 is widely used in agriculture, but the mechanisms of Rhg1 disease resistance have remained unclear.
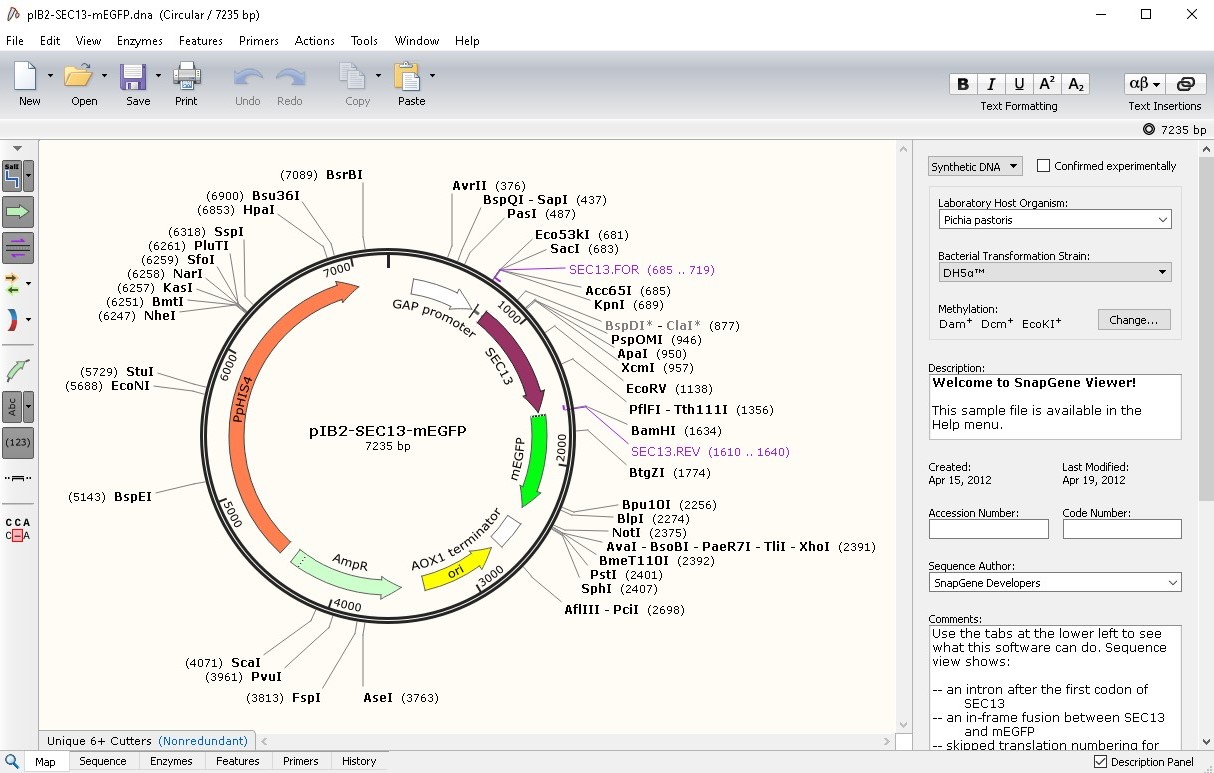
glycines), the most economically damaging pathogen of soybeans worldwide. These Rhg1 loci mediate resistance to soybean cyst nematode (SCN H. However, certain haplotypes of the Rhg1 (resistance to Heterodera glycines 1) locus of soybean possess multiple repeat copies of an α-SNAP gene ( Glyma.18G022500) that encodes atypical amino acids at a highly conserved functional site. Α-SNAP and NSF proteins are conserved across eukaryotes and sustain cellular vesicle trafficking by mediating disassembly and reuse of SNARE protein complexes, which facilitate fusion of vesicles to target membranes.
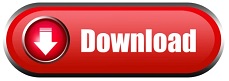